Design Oriented Analysis of the Two-Output Isolated Soft-Switching PFC Circuit
|
|
Boris Sasic
KEPCO, Inc., Engineering Dept.
Abstract: This paper deals with a power factor correction circuit based on the isolated boost
converter. An additional circuit provides zero-voltage-transition (ZVT) switching while
maintaining constant frequency operation. A transformer used to provide galvanic isolation
enables a wide range of output voltages, either lower or higher than the input voltage, allowing
multi-output solutions. By optimizing the transformer turns ratio, output voltage(s) can be
adjusted to optimum value for any postregulator circuit (e.g., buck or buck-boost). This means
that very high overall efficiency can be achieved with excellent regulation and fast response.
After a brief analysis of the isolated ZVT boost converter and the two-output version, one way
to optimize transformer turns ratio and a solution for control circuitry are proposed. Finally,
advantages and disadvantages are discussed, and possible applications as a single converter,
without postregulators, are also considered.
|
|
|
1. INTRODUCTION
Power factor correction (PFC) circuits have become standard input stages for almost every
medium and high power switching power supply operating at the mains voltage. Today's most
often used configuration includes a boost converter as an input stage, stabilizing the input to the
second stage at a level 10-20% over the maximum amplitude of mains voltage. The second stage
is usually a half- or full-bridge converter which provides all necessary output voltages.
Efficiency of the boost PFC is high (90-95% under favorable conditions), but the second stage
converter has a maximum efficiency of about 85% (which is significantly reduced if high output
currents are produced, down to about 70%). Overall maximum efficiency is, therefore, about
80%.
Several years ago resonant converters were introduced as a way to increase efficiency and reduce
radiated and conducted noises. Unfortunately, high circulating current has prevented their use
at higher power levels. Many soft-switching configurations were developed in an effort to reduce
component stress, although these designs continued to retain variable frequency operation (and
associated EMI/RFI problems).
Soft-switching techniques recently developed eliminate variable frequency operation, further
reducing component stress and improving efficiency. Zero-voltage and zero-current switching
(ZVS and ZCS) modifications of almost every basic converter topology have been widely
accepted, and the semiconductor industry has followed that trend by developing several fully
integrated controllers. The full-bridge, phase-shift ZVS topology is the most frequently used [1],
[2].
2. ZVT TECHNIQUE
One of the latest soft-switching techniques was referred to by its designers as the Zero-Voltage-Transition (ZVT) technique [3]. By implementing an additional auxiliary network consisting of
an inductor, capacitor and one switch, full zero-voltage switching is enabled for both main
switches and rectifier diodes. Boost converters are very suitable for ZVT applications either in
basic (nonisolated) or isolated versions. The boost converter modified for ZVT operation is
shown in Fig.1.

FIG. 1. ZVT Boost converter
It differs from a conventional boost PWM converter by possessing an additional network
consisting of a resonant inductor (Lr), an auxiliary switch (Qa), and a diode (Da). Cr is the
resonant capacitor, which incorporates the output capacitance of the power switch and the
junction capacitance of the rectifier. Detailed description of circuit operation is given in [3] and
[4].
3. ISOLATED ZVT BOOST
By combining benefits of the boost topology and ZVT technique, efficiency of the PFC circuits
can be even higher than in the classic approach. Adding a transformer for galvanic isolation opens
some very interesting possibilities, such as: the output voltage can be either higher or lower than
amplitude of the mains voltage; improved safety; simpler converters (which, in turn, means more
efficient) can be used for additional output voltage regulation; multiple output configurations are
easily achievable; and, in certain cases, using only the PFC converter will be enough to provide
power of the desired quality.
The more complex topology of such circuits means they tend to be used more often in converters
requiring higher power. The full-bridge configuration, presented in Fig.2, is best for high input
currents and high input voltages. When intended for use with a lower input voltage (e.g., 115V
mains) and/or lower output power, a full-bridge form can be replaced by the two-transistor
version, simplifying the circuit, but with the expense of doubled maximum voltage across the
switches.

FIG. 2. Full-bridge ZVT boost converter
By using a transformer, output voltage can be set to a value lower than the amplitude of the
mains voltage. Along with galvanic isolation, it provides additional safety, allowing a more
comfortable choice of the rectifying diodes, output capacitors and switches if the postregulator
circuit is used. Multiple outputs are easily achievable when desired for specific applications.
Fig.2. shows a two-output version, where the first output (Vo1) is intended for higher current,
while the second output has lower current/higher voltage. Split secondary windings used for the
first output enable the use of thinner wire; this, and the use of only two diodes, reduce power
loss and voltage drop. Even further loss reduction can be achieved by using synchronous
rectifiers.
4. CIRCUIT ANALYSIS
Circuit shown in Fig.2. operates in similar manner as that from Fig.1. Only difference is
implementation of the phase-shift control with overlapping MOSFETs on times in order to
provide a path for inductor charging current.
Waveforms presented in Fig.3 show the pattern for driving the switches, together with the
associated choke current. The input choke operates with doubled switching frequency, similar
to other full-bridge converters. Since soft-switching enables a significant increase in the operating
frequency, this further reduces dimensions of the input choke as compared to standard boost
converters.

FIG. 3. Driving waveforms and choke current
The basic equations defining this circuit are derived by following standard and simple procedures.
The most important equation defines the output-to-input voltage ratio:
where,
- output voltage,
- input voltage,
n - transformer turns ratio,
- duty-cycle.
Since the input choke is also charging during a part of the soft-switching transition (when voltage
across the Q1 falls to zero), the duty-cycle is extended for:
where the meaning of each variable is given in Fig.2 and 3.
Solving equation (1), the term for maximum voltage across open switches (VSW) is derived:
This voltage depends on the duty-cycle, so for minimum Vsw , the minimum value of the duty-cycle should be chosen. As can be seen from the waveforms in Fig.1,
is zero when the duty-cycle of the switching transistors reaches 50%, so the minimum value of
due to the on and off
times of the switches is of no concern. However, a lower
means a need for lower input
inductance (as can be shown analytically), and a consequently higher input current ripple.
Defining maximum allowable ripple allows
min to be calculated. By adjusting this calculated
value to a practical value, then plugging it into equation (1) using a known output voltage, the
transformer turns ratio can be found.
5. CONTROL CIRCUIT
At this time, there are no integrated controllers available for this purpose; only single-output PFC
integrated circuits can be found. Fortunately, the proposed driving pattern can be achieved using
a standard PFC controller and just a few additional ICs. Although peak current mode control is
possible, for better results we have based control electronics around the UC3854 with average
current mode operation implemented [5], [6]. Output from the PFC integrated controller is fed
into circuit shown in Fig.4. (signal PWM0).

FIG. 4. Control circuit
This circuit provides driving signals for four main and one auxiliary switches. Retriggerable
monostable multivibrator (U4) is used for precise definition of Qa's on time. Waveforms shown
in Fig.5. further explains circuit operation.

FIG. 5. Control circuit waveforms
6. SIMULATION RESULTS
Computer simulation of the full-bridge boost converter power stage was used for detailed
analysis of the circuit. The main focus of the analysis was to explore using the parasitic
capacitances of the power switches to replace Cr. When the resonant capacitance Cr was
distributed across the power MOSFETs, no negative influence was observed. The current stress
of the resonant capacitor was significantly reduced; in some applications, additional capacitance
can be completely omitted, reducing the component count. Simulation waveforms are shown in
Figs.6 and 7.
For reason of clarity, resonant frequency of the Lr, Cr network is lower than it should be, so
transient times are longer. Thus, soft switching transitions for both switches and rectifiers are
emphasized.
Additional components (compared to a standard boost converter) have an inverse effect on
efficiency. On the other hand, lower voltage stress enables use of MOSFETs with lower RDS(on)
and Schottky diodes. Soft-switching eliminates switching losses for both switches and rectifiers.
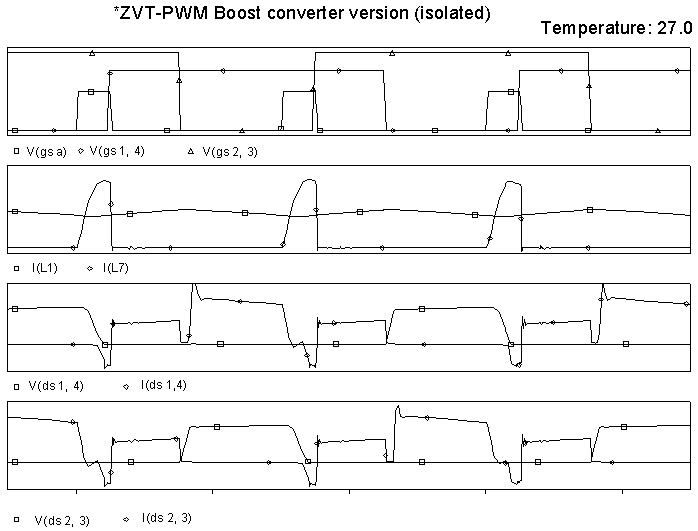
FIG. 6. Simulation waveforms

FIG. 7. Diode current and voltage
7. FIELDS OF APPLICATION
Although somewhat lower than for standard boost, efficiency is still comparable and in the 90%
range. For many applications output voltage stability and transient response will be good enough
without the need for additional converters, so that overall efficiency will be much higher than in
standard configurations. Even for demanding applications, such as those requiring high stability
and a fast transient response, a simple buck or buck-boost converter (having very low losses) can
be added, allowing a high overall efficiency to be maintained..
A significant advantage of the isolated version is the ability to allow short-circuit protection,
which is impossible for the standard, nonisolated boost converter. This makes real redundant
operation of paralleled converters possible with a single PFC, the trend currently being observed
in modern switching supplies.
The main disadvantage of this configuration is that transformer leakage inductance is not utilized;
however, the same problem also occurs with some of the other ZVS techniques. This means that
the transformer should be designed for minimum leakage. By applying the interleaving technique,
leakage inductance can be minimized at the cost of increased winding capacitances; these
capacitances, however, become part of the resonant capacitance and do not cause any problems.
8. CONCLUSION
PFC circuits have their place in almost every medium and high power switching supply. Many
national standards tend to define the use of the PFC as practically obligatory at lower power
levels. With that in mind, today's "unity power factor" power supplies, consisting of two
separate converters, present many disadvantages. Soft-switched, galvanically isolated PFC
converters, however, offer the possibility of multiple outputs, which should prove very useful for
many applications. At high power levels, their higher overall efficiency also plays a significant
role. For distributed power supply systems, bus voltage produced without a postconverter means
lower heating and higher reliability. In addition, low EMI/RFI makes it suitable for
telecommunications, as well as for industrial applications.
References
[1] Andreycak, B. : "Phase Shifted, Zero Voltage Transition Design Considerations and the UC3875 PWM Controller," Application note U-136, Unitrode Databook 1995/96.
[2] Hamo, D. J.: "A 50W, 500kHz, Full-Bridge, Phase-Shift, ZVS Isolated DC to DC Converter Using the HIP4081A," Application note 9506, Harris, 1995.
[3] Hua, G.; Lee, F. C.: "An Overview of Soft Switching Techniques for PWM Converters," EPE Journal, Vol. 3, March 1993.
[4] Noon, J. P.: "A 250kHz, 500W Power Factor Correction Circuit Employing Zero Voltage Transitions," Switching Regulated Power Supply Design Manual, Unitrode, 1995.
[5] Todd, P. C.: "UC3854 Controlled Power Factor Correction Circuit Design," Application note U-134, Unitrode Databook1995/96.
[6] Dixon, L. : "Average Current Mode Control of Switching Power Supplies," Application note U-140, Unitrode Databook 1995/96.
** This paper was originally published in the Proceedings of the International Power Conversion Conference, Nürnberg, Germany, May 21-23, 1996; pp. 429-437